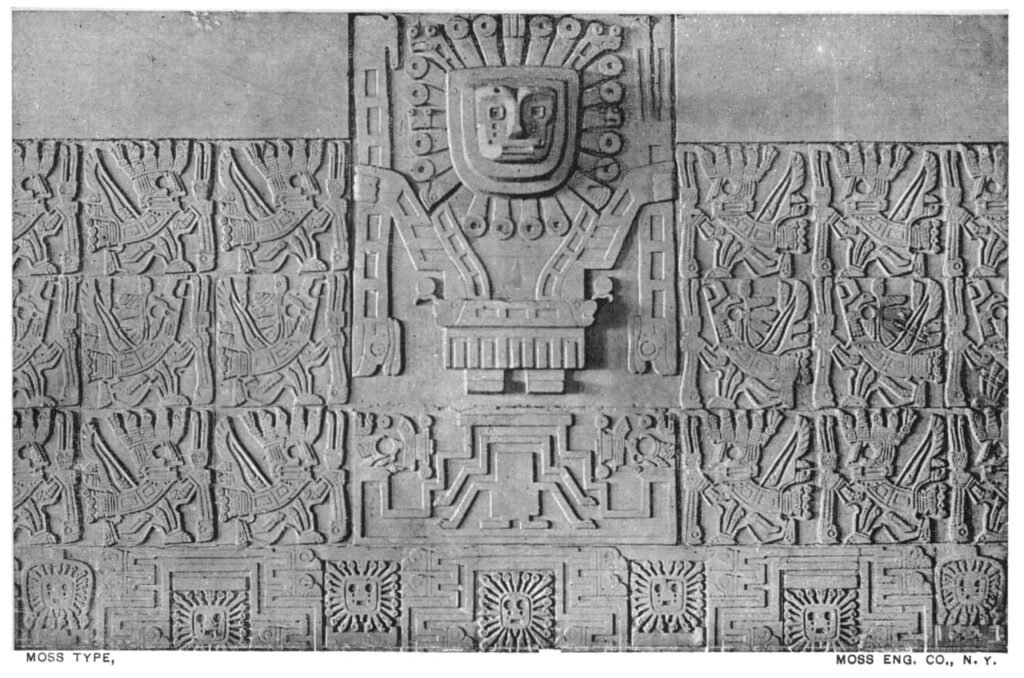
The fascinating world of dwarf planets and their connection to plate tectonics has captivated scientists and space enthusiasts alike. One of the most intriguing aspects of this topic is the tilted axial orientation of Uranus and its relation to the plane of the ecliptic. Additionally, the classification of other celestial bodies such as Pluto, Ceres, Haumea, Makemake, and Eris as dwarf planets further deepens our understanding of the vast and diverse universe we inhabit.
Uranus and its Tilted Axial Orientation
The planet Uranus stands out among its siblings in the solar system due to its unique axial tilt. Unlike most planets, whose axes of rotation are nearly perpendicular to the plane of the ecliptic, Uranus rotates on its side, with its axis tilted almost parallel to the plane of the ecliptic.
This peculiar orientation has puzzled astronomers for centuries. The discovery of Uranus’s axial tilt was made in 1781 by William Herschel, who noted the pronounced deviation of the planet’s equator from its orbital path. This distinctive feature sets Uranus apart from all other known planets and has sparked extensive scientific research into the mechanisms that govern its rotational characteristics.
Data and statistics gathered from multiple missions and observations reveal that Uranus’s axial tilt is approximately 98 degrees. This means that the planet lies almost horizontally on its side as it orbits around the Sun. The exact cause of this extreme tilt remains a subject of study and debate among scientists.
The Classification of Dwarf Planets
In the past, the definition of a planet seemed relatively straightforward. However, as our knowledge of the solar system expanded, astronomers realized that Pluto and some other celestial bodies did not fit the traditional criteria for being considered full-fledged planets.
Pluto, originally designated as the ninth planet in our solar system, was reclassified as a dwarf planet in 2006 by the International Astronomical Union (IAU). The decision came after much deliberation and debate within the scientific community. The IAU established three criteria for a celestial body to be classified as a planet:
- It must orbit the Sun.
- It must be spherical in shape due to its own gravitational forces.
- It must have cleared its orbit of other debris.
Based on these criteria, Pluto was deemed ineligible for full planet status, as it shares its orbit with other celestial bodies in the Kuiper Belt. Instead, Pluto was reclassified as a dwarf planet, along with other objects such as Ceres, Haumea, Makemake, and Eris. These five celestial bodies meet the first two criteria of being spherical and orbiting the Sun but fail to meet the third criterion of clearing their orbits.
Pluto: The Once Ninth Planet
Pluto’s history as the ninth planet in our solar system is an integral part of understanding the significance of its reclassification as a dwarf planet. Discovered in 1930 by Clyde Tombaugh, Pluto enjoyed the status of a planet for more than seven decades.
However, advancements in technology and observations brought to light the existence of a vast region beyond Neptune known as the Kuiper Belt. This region is populated by small bodies, including Pluto. The discovery and increasing knowledge of the Kuiper Belt presented a challenge to the long-established definition of a planet.
After its reclassification, Pluto became the prototypical example of a dwarf planet. It is a fascinating object located in the outer regions of our solar system, and studying it provides valuable insights into the formation and evolution of our celestial neighborhood.
Ceres: The Largest Object in the Asteroid Belt
Ceres, the largest object in the asteroid belt between Mars and Jupiter, stands out as a remarkable dwarf planet. Discovered in 1801 by Italian astronomer Giuseppe Piazzi, Ceres has been the subject of extensive study and exploration in recent years.
Ceres is unique in that it is not only a dwarf planet but also the largest known object in the asteroid belt. It is essentially a rocky body with a diameter of approximately 590 miles (940 kilometers) and a mass that accounts for about a third of the total mass of the asteroid belt.
The composition of Ceres is one of its most intriguing aspects. It is composed of a rocky core covered by a thick layer of ice and possibly a subsurface ocean. Observations by NASA’s Dawn spacecraft, which orbited Ceres from 2015 to 2018, have revealed numerous geological features, including mountains and crater-like formations.
The study of Ceres provides valuable insights into the origin and evolution of the inner solar system, as well as the processes that shaped the early stages of planetary formation.
Haumea: A Peculiarly Shaped Dwarf Planet
Haumea is a dwarf planet located in the outer regions of the solar system beyond Neptune. Discovered in 2004 by a team of Spanish astronomers, Haumea is notable for its peculiar elongated shape.
Unlike most dwarf planets, which have a roughly spherical shape, Haumea has an elongated shape resembling a rugby ball. This unique morphology is believed to be the result of a high rotational speed, causing the planet to become elongated along its equatorial plane.
The elliptical shape of Haumea has led to speculation that it may have experienced a collision with another celestial body in the past. This collision would have disrupted the planet’s original form, resulting in its current elongated shape.
Makemake: The Fourth Largest Dwarf Planet
Makemake, named after the creation deity of the Rapa Nui people of Easter Island, is another intriguing member of the dwarf planet family. It was discovered in 2005 by a team of astronomers led by Michael E. Brown.
Makemake is the fourth largest known dwarf planet, with a diameter of approximately 880 miles (1,400 kilometers). It is located in the Kuiper Belt, a region of the solar system beyond the orbit of Neptune.
One of the notable features of Makemake is its relatively high albedo, which is the measure of the reflectivity of an astronomical body. The surface of Makemake is composed mainly of frozen methane and nitrogen, giving it a brilliant white appearance when viewed from a distance.
Makemake’s atmosphere is extremely thin, consisting primarily of nitrogen, with possible traces of methane. Studying the composition and characteristics of Makemake provides invaluable insights into the volatile dynamics of the outer regions of the solar system.
Eris: The Most Massive Dwarf Planet
Eris, named after the Greek goddess of strife and discord, is the most massive known dwarf planet in our solar system. It was discovered in 2005 by a team of astronomers led by Michael E. Brown.
Eris is located in the Kuiper Belt and has a diameter of approximately 1,445 miles (2,326 kilometers), making it slightly smaller than Pluto. Despite its size, it has a higher mass compared to Pluto.
One of the defining characteristics of Eris is its highly elliptical orbit, which takes it far beyond the orbit of Pluto at its farthest point from the Sun. Its distance from the Sun and its slow orbital speed make it one of the coldest known objects in the solar system.
Eris’s surface is believed to be composed of a mixture of rocky material and frozen gases such as methane and nitrogen. The presence of these volatile substances suggests that the planet has undergone significant geological activity over its lifetime.
Plate Tectonics: The Earth’s Dynamic Crust
Plate tectonics is a fundamental concept in the field of Earth and space science, providing a comprehensive framework for understanding the dynamic nature of our planet’s crust.
The theory of plate tectonics proposes that the Earth’s lithosphere, which is composed of several large and small plates, floats on the underlying asthenosphere. These plates are in constant motion, driven mainly by heat from within the Earth, resulting in various geological phenomena such as earthquakes, volcanoes, and the formation of mountain ranges.
The Theory of Plate Tectonics
The theory of plate tectonics is built upon numerous scientific observations and concepts. It explains how the Earth’s lithosphere is broken into several distinct plates that interact with each other along their boundaries.
The primary types of plate boundaries are:
- Divergent boundaries: where plates move away from each other, creating new crust.
- Convergent boundaries: where plates collide, resulting in the formation of mountain ranges, volcanic arcs, and subduction zones.
- Transform boundaries: where plates slide past each other horizontally, leading to significant earthquakes.
These boundaries are critical zones where various geological features and processes occur, offering valuable insights into the mechanisms behind plate tectonics.
Evidence Supporting Plate Tectonics
Multiple lines of evidence support the theory of plate tectonics, showcasing the remarkable interconnectedness of geological processes across the Earth’s surface:
Forms of Relief
The distribution of mountain ranges, coastline alignments, and deep-sea trenches provides evidence for plate tectonics. For example, the presence of significant mountain ranges along subduction zones, such as the Andes and the Cascade Range, supports the idea of convergent plate boundaries.
Earthquakes
Earthquakes are often located along plate boundaries, demonstrating the active nature of plate tectonics. For instance, the Ring of Fire, a region in the Pacific Ocean known for its intense seismic activity, is a direct consequence of the interactions between several tectonic plates.
Volcanoes
Volcanoes also provide evidence for plate tectonics, particularly at convergent and divergent boundaries. The presence of volcanic arcs, such as the Pacific Ring of Fire and the Mediterranean volcanic arc, highlights the connection between plate boundaries and volcanic activity.
These are just a few examples of the evidence supporting plate tectonics. Collectively, these observations provide substantial proof of the dynamic and interconnected nature of our planet’s geological processes.
Seafloor Spreading and Mid-Ocean Ridges
A particularly compelling piece of evidence for plate tectonics is seafloor spreading. This process occurs at divergent plate boundaries, where two plates move away from each other, allowing magma to rise and form new oceanic crust.
As the molten material solidifies, it creates a new section of seafloor, pushing the older crust away from the ridge. This continuous process of seafloor spreading results in the formation of long underwater mountain ranges known as mid-ocean ridges.
One notable example of a mid-ocean ridge is the Mid-Atlantic Ridge, which stretches from the Arctic Ocean to the southernmost tip of Africa. The formation of these ridges provides compelling evidence for the movement and interactions of tectonic plates.
Subduction Zones and Volcanic Arcs
Another crucial aspect of plate tectonics is the presence of subduction zones, where one tectonic plate is forced beneath another. These subduction zones often give rise to volcanic activity and the formation of volcanic arcs.
A prime example of a volcanic arc is the Aleutian Arc, located in southwestern Alaska. It is a prime location for the study of plate tectonics, as it represents the convergence of the Pacific Plate and the North American Plate.
Implications and Importance of Plate Tectonics
Understanding plate tectonics is crucial for various scientific disciplines, as it has wide-ranging implications for fields such as geology, climatology, and the study of both past and present life on Earth.
The movement of tectonic plates has shaped the Earth’s continents over millions of years, leading to the formation of mountains, valleys, and other landforms. It has influenced patterns of weather and climate, redistributing heat and moisture across the planet.
Plate tectonics also plays a pivotal role in the evolution of life on Earth. The movement of continents has affected habitats, isolated populations, and provided opportunities for the evolution and diversification of species.
Ultimately, the study of dwarf planets and their connection to plate tectonics offers a unique perspective on the complexity and interconnectedness of the celestial bodies in our universe. By unraveling the mysteries of these celestial objects and the forces that shape our planet, scientists take important steps towards unlocking the secrets of our cosmic neighborhood.
Conclusion
In conclusion, the world of dwarf planets provides endless scientific exploration and understanding. We have delved into the uniqueness of Uranus and its remarkably tilted axis, as well as the classification of dwarf planets like Pluto, Ceres, Haumea, Makemake, and Eris. Each of these celestial bodies has its own distinctive characteristics that contribute to our understanding of the vastness and diversity of our solar system.
Additionally, the study of plate tectonics further enhances our comprehension of the dynamic nature of the Earth’s crust. The theory of plate tectonics, supported by an array of evidence, enables scientists to interpret and predict geological phenomena such as earthquakes, volcanoes, and the formation of mountain ranges.
By studying both dwarf planets and plate tectonics, we gain invaluable insights into the universe we inhabit. These remarkable fields of study, interconnected in their own right, not only expand our knowledge but also deepen our appreciation for the complex systems and processes that shape our planet and the celestial bodies beyond.
As we continue on our journey of exploration and understanding, the significance of comprehending and studying these concepts becomes ever more apparent. Just as the Mayan Morning Star brings forth the light of knowledge, so too do the discoveries and insights gained through the study of dwarf planets and plate tectonics illuminate the wonders of the universe.